Microbiology has had a long, rich history,
initially centered in the causes of infectious diseases but now including
practical applications of the science. Many individuals have made significant
contributions to the development of microbiology.
Early history of microbiology. Historians
are unsure who made the first observations of microorganisms, but the
microscope was available during the mid‐1600s, and an English scientist namedRobert
Hooke made key observations. He is reputed to have observed strands of
fungi among the specimens of cells he viewed. In the 1670s and the decades
thereafter, a Dutch merchant namedAnton van Leeuwenhoek made
careful observations of microscopic organisms, which he called animalcules. Until
his death in 1723, van Leeuwenhoek revealed the microscopic world to scientists
of the day and is regarded as one of the first to provide accurate descriptions
of protozoa, fungi, and bacteria.
After van Leeuwenhoek died, the study of
microbiology did not develop rapidly because microscopes were rare and the
interest in microorganisms was not high. In those years, scientists debated the
theory of spontaneous generation, which stated that microorganisms
arise from lifeless matter such as beef broth. This theory was disputed
by Francesco Redi, who showed that fly maggots do not arise from
decaying meat (as others believed) if the meat is covered to prevent the entry
of flies. An English cleric named John Needham advanced
spontaneous generation, but Lazzaro Spallanzanidisputed the theory
by showing that boiled broth would not give rise to microscopic forms of life.
Louis Pasteur and the germ theory. Louis
Pasteur worked in the middle and late 1800s. He performed
numerous experiments to discover why wine and dairy products became sour, and
he found that bacteria were to blame. Pasteur called attention to the
importance of microorganisms in everyday life and stirred scientists to think
that if bacteria could make the wine “sick,” then perhaps they could cause
human illness.
Pasteur had to disprove spontaneous
generation to sustain his theory, and he therefore devised a series of swan‐necked flasks filled with broth. He left the flasks
of broth open to the air, but the flasks had a curve in the neck so that
microorganisms would fall into the neck, not the broth. The flasks did not
become contaminated (as he predicted they would not), and Pasteur's experiments
put to rest the notion of spontaneous generation. His work also encouraged the
belief that microorganisms were in the air and could cause disease. Pasteur
postulated the germ theory of disease, which states that
microorganisms are the causes of infectious disease.
Pasteur's attempts to prove the germ theory
were unsuccessful. However, the German scientist Robert Koch provided
the proof by cultivating anthrax bacteria apart from any other type of
organism. He then injected pure cultures of the bacilli into mice and showed
that the bacilli invariably caused anthrax. The procedures used by Koch came to
be known as Koch's postulates (Figure ). They provided a
set of principles whereby other microorganisms could be related to other
diseases.
The development of microbiology. In
the late 1800s and for the first decade of the 1900s, scientists seized the
opportunity to further develop the germ theory of disease as enunciated by
Pasteur and proved by Koch. There emerged a Golden Age of Microbiology during
which many agents of different infectious diseases were identified. Many of the
etiologic agents of microbial disease were discovered during that period,
leading to the ability to halt epidemics by interrupting the spread of
microorganisms.
Despite the advances in microbiology, it was
rarely possible to render life‐saving therapy to an infected patient. Then,
after World War II, the antibiotics were introduced to
medicine. The incidence of pneumonia, tuberculosis, meningitis, syphilis, and
many other diseases declined with the use of antibiotics.
Work with viruses could not be effectively
performed until instruments were developed to help scientists see these disease
agents. In the 1940s, theelectron microscope was developed and
perfected. In that decade, cultivation methods for viruses were also
introduced, and the knowledge of viruses developed rapidly. With the
development of vaccines in the 1950s and 1960s, such viral diseases as polio,
measles, mumps, and rubella came under control.
Modern microbiology. Modern
microbiology reaches into many fields of human endeavor, including the
development of pharmaceutical products, the use of quality‐control methods in food and dairy product production, the
control of disease‐causing microorganisms in consumable waters,
and the industrial applications of microorganisms. Microorganisms are used to
produce vitamins, amino acids, enzymes, and growth supplements. They
manufacture many foods, including fermented dairy products (sour cream, yogurt,
and buttermilk), as well as other fermented foods such as pickles, sauerkraut,
breads, and alcoholic beverages.
One of the major areas of applied
microbiology is biotechnology. In this discipline,
microorganisms are used as living factories to produce pharmaceuticals that
otherwise could not be manufactured. These substances include the human hormone
insulin, the antiviral substance interferon, numerous blood‐clotting factors and clotdissolving enzymes, and a number
of vaccines. Bacteria can be reengineered to increase plant resistance to
insects and frost, and biotechnology will represent a major application of
microorganisms in the next century.
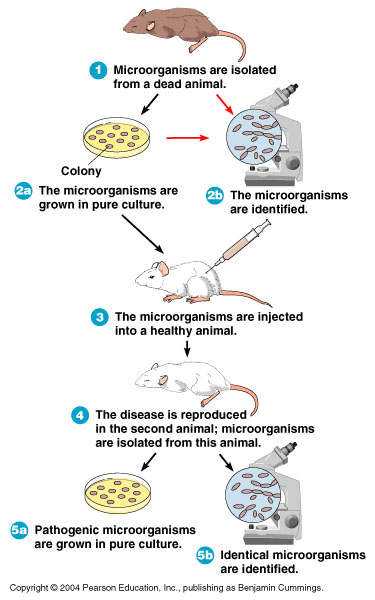
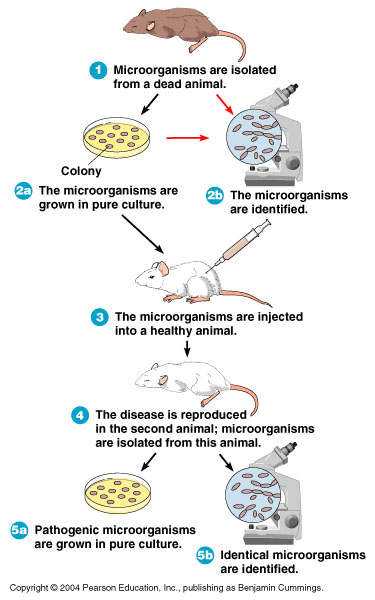
The steps of Koch's postulates used to relate
a specific microorganism to a specific disease. (a) Microorganisms are observed
in a sick animal and (b) cultivated in the lab. (c) The organisms are injected
into a healthy animal, and (d) the animal develops the disease. (e) The
organisms are observed in the sick animal and (f) reisolated in the lab.
Work
with viruses could not be effectively performed until instruments were
developed to help scientists see these disease agents. In the 1940s, the electron
microscopewas developed and perfected. In that decade, cultivation methods
for viruses were also introduced, and the knowledge of viruses developed
rapidly. With the development of vaccines in the 1950s and 1960s, such viral
diseases as polio, measles, mumps, and rubella came under control
https://biologysciencesonline.blogspot.com/2019/04/types-of-microbiology-major-groups-of.html
https://biologysciencesonline.blogspot.com/2019/04/types-of-microbiology-major-groups-of.html